Synergistic science
Besides focusing on process engineering, food engineers need to become product engineers who interact with other scientific disciplines to better understand food at the nano- and micro-scale level in order to fabricate more nutritious, flavorful and transportable products, argues José Miguel Aguilera.
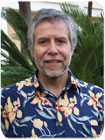
Aguilera is a professor in the Department of Chemical and Bioprocess Engineering at Universidad Católica de Chile in Santiago, Chile. He also is this year's recipient of the Marcel Loncin Research Prize from the Institute of Food Technologists, which presents the biennial award and a $50,000 stipend to support basic research. Aguilera will use the funds to extract numerical data on materials such as the surface roughness of chocolate bars from microscopy images and apply algorithms for use in engineering modeling and product design.
After receiving a BS degree in chemical engineering from Pontificia Universidad Católica, Aguilera pursued an MBA from Texas A&M University and a MS from Massachusetts Institute of Technology. He also earned a PhD in food science from Cornell University. Aguilera is a fellow at the Nestle Research Center in Lucerne, Switzerland.
Most approaches to food manufacturing have roots in chemical engineering, he maintains, but little is understood about how food matrices are formed during processing. By quantifying the microstructure formations of foods, engineers will be better able to control their transport properties, texture, surface color and nutritional properties. Aguilera calls this approach food material science.
FE: Define food material science.
Aguilera: In the past, food engineers were constrained by a trial-and-error approach to the design of products. Now they can tap a tremendous body of knowledge. At Nestle, for example, there is the food microstructure group, which has about 15 scientists with expertise in specialized areas such as colloid chemistry and polymer science. Unilever has a similar group. When experts in areas like insoluble fibers, emulsions and the functionality of specific proteins work together, I call it food material science (FMS).
It is not enough to work with other specialists in food; food engineers must collaborate with and learn from specialists in other disciplines. Sometimes we isolate ourselves to protect status-quo thinking. FMS will change empirical development of food fabrication processes to a more systematic approach.
More collagen-binding factors are present in tough meat, just as stale bread has more retrograded starch and bloomed chocolate has more cocoa butter migration to the surface. If we look at these foods' microstructure, we see that it relates to their functionality. FMS provides the link between how molecules interact at the molecular level, the type of assemblies they form, and the fat droplets, air bubbles and other microstructural elements present that affect their ultimate appearance and taste.
FE: You have noted the trend toward more convenient and healthy foods. How does FMS contribute to this?
Aguilera: Much has been learned about the structure of foods and bioavailability of nutrients. Nature places nutrients in specific matrices, and the release of those nutrients is further affected by structures formed during processing. The pro-vitamin A carotenes in carrots are associated with reduced risk of heart disease and certain types of cancer. However, how the body releases carotene from fruits and vegetables is just beginning to be understood. If a raw carrot is chewed, only 15%-20% of the carotene is available in our blood. On the other hand, if a carrot is destroyed and converted to juice, 95% of the carotene is available. The nutritional value is unleashed by altering cell structure for human digestion.
FE: Name some examples of the power of collaborative science. Aguilera: Three of my PhD students have spent from two to five months working with Professor Christopher Brown, a mechanical engineer at Worcester Polytechnic Institute in Massachusetts. Christopher is a scientist who uses a scanning laser microscope to analyze, for example, the roughness of aircraft runways for NASA. With his help, we're analyzing the roughness of food.
One of my students was working on a project involving spaghetti. He wanted to study the submicroscopic roughness of various samples to determine why sauce clings better to some. The student learned about Christopher Brown's work with the scanning laser microscope, and Christopher invited him to come to his lab. They found that spaghetti's functional performance is affected by the extruder die. Many manufacturers have switched to Teflon-coated dies to reduce dough friction and improve extruder throughput. But the spaghetti's surface, it turns out, becomes very smooth, and sauce won't cling to it as it would if a brass die is used. The gloss and color also are affected.
Surface texture has a tremendous impact on all types of foods. The roughness of a French fry influences the amount of oil that is absorbed during frying, for example. Oil uptake occurs at a micron level, and unless advanced microscopy is applied to analyze the surface texture, there is no way for a food engineer to know what the performance will be.
FE: So a Teflon-coated cutting surface could produce a healthier French fry that also is less expensive to fry because of reduced oil pickup.
Aguilera: That's right. Most oil inside the crust is sucked from the surface as the fries are removed from the fryer. Drainage would be facilitated and less oil would cling to cuts that are smoother in the micron range.
FE: Have you used a scanning laser microscope in your confectionery research?
Aguilera: Yes. After acquiring the images, we have applied fractal analysis to quantify the roughness of bloomed chocolate and other surfaces. Fractal analysis puts a number to complex geometries to develop a map of the exact kinetics at various temperatures and surface roughness. The geometric profile of a bloomed chocolate is not a straight line but a series of hills and valleys. I don't know if we'll ever develop a reduced calorie chocolate that tastes exactly like regular chocolate, but unless we know how the structure of chocolate is made and destroyed in the mouth, we're doomed to spend years on ingredient trial and error.
FE: What are some other instances where a better understanding of the microstructure of food impacts processing?
Aguilera: Artificial caviar is a concept that was patented many years ago but never successful commercially because of costs. But chefs are finding they can create artificial caviar by making a solution of a gelling polymer, adding flavor and colors and then using a syringe-type die to make droplets that form little balls of gel when they contact a calcium solution.
FE: Are you suggesting that the processing tools used today are inappropriate?
Aguilera: Nobody hammers a nail with a bulldozer, but often the dimensions of the key microstructural elements of a food are grossly out of proportion to the size of the device used to produce it. We make droplets in emulsions by dissipating a lot of energy in a 1 millimeter gap, but the structural element is only 10 microns, 100 times smaller. This will change. We are starting to use membranes with pores comparable to emulsion droplets, just as the cow does with milk.
FE: To what extent is the shift from a process engineering focus to product engineering occurring?
Aguilera: At the 2004 International Congress of Engineering and Food, thermal processing, mass transfer and other traditional approaches to food engineering were the focus of many of the papers, but the most popular topics were processing and characterization of structured polyphasic systems. These are complex, multicomponent mixtures with many phases, such as ice cream, which has fat, water, sugar, and at least three phases: solid ice, liquid sugar solution and gas air bubbles. Structured polyphasic systems weren't even being discussed at the first Congress in 1974.
Interfaces are occurring between scientific and culinary communities that never interacted before. Chefs are using centrifuges to create sophisticated new foods. Molecular biologists are creating tomatoes with stronger cell walls that can yield better paste. We're developing interfaces with the pharmaceutical industry for controlled release of flavors and nutritionals. By developing relationships with nutritionists, material scientists and others, we can design products to meet new consumer demands.
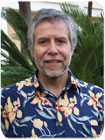
Professor José Miguel Aguilera, Universidad Católica de Chile, Santiago.
Aguilera is a professor in the Department of Chemical and Bioprocess Engineering at Universidad Católica de Chile in Santiago, Chile. He also is this year's recipient of the Marcel Loncin Research Prize from the Institute of Food Technologists, which presents the biennial award and a $50,000 stipend to support basic research. Aguilera will use the funds to extract numerical data on materials such as the surface roughness of chocolate bars from microscopy images and apply algorithms for use in engineering modeling and product design.
After receiving a BS degree in chemical engineering from Pontificia Universidad Católica, Aguilera pursued an MBA from Texas A&M University and a MS from Massachusetts Institute of Technology. He also earned a PhD in food science from Cornell University. Aguilera is a fellow at the Nestle Research Center in Lucerne, Switzerland.
Most approaches to food manufacturing have roots in chemical engineering, he maintains, but little is understood about how food matrices are formed during processing. By quantifying the microstructure formations of foods, engineers will be better able to control their transport properties, texture, surface color and nutritional properties. Aguilera calls this approach food material science.
FE: Define food material science.
Aguilera: In the past, food engineers were constrained by a trial-and-error approach to the design of products. Now they can tap a tremendous body of knowledge. At Nestle, for example, there is the food microstructure group, which has about 15 scientists with expertise in specialized areas such as colloid chemistry and polymer science. Unilever has a similar group. When experts in areas like insoluble fibers, emulsions and the functionality of specific proteins work together, I call it food material science (FMS).
It is not enough to work with other specialists in food; food engineers must collaborate with and learn from specialists in other disciplines. Sometimes we isolate ourselves to protect status-quo thinking. FMS will change empirical development of food fabrication processes to a more systematic approach.
More collagen-binding factors are present in tough meat, just as stale bread has more retrograded starch and bloomed chocolate has more cocoa butter migration to the surface. If we look at these foods' microstructure, we see that it relates to their functionality. FMS provides the link between how molecules interact at the molecular level, the type of assemblies they form, and the fat droplets, air bubbles and other microstructural elements present that affect their ultimate appearance and taste.
FE: You have noted the trend toward more convenient and healthy foods. How does FMS contribute to this?
Aguilera: Much has been learned about the structure of foods and bioavailability of nutrients. Nature places nutrients in specific matrices, and the release of those nutrients is further affected by structures formed during processing. The pro-vitamin A carotenes in carrots are associated with reduced risk of heart disease and certain types of cancer. However, how the body releases carotene from fruits and vegetables is just beginning to be understood. If a raw carrot is chewed, only 15%-20% of the carotene is available in our blood. On the other hand, if a carrot is destroyed and converted to juice, 95% of the carotene is available. The nutritional value is unleashed by altering cell structure for human digestion.
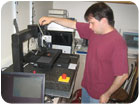
Brendan Powers, manager of the Surface Metrology Lab at Worcester Polytechnic Institute, utilizes the lab's scanning laser microscope. The lab is helping engineers gain a better understanding of processed foods on a nano-scale. Source: Worcester Polytechnic Institute.
One of my students was working on a project involving spaghetti. He wanted to study the submicroscopic roughness of various samples to determine why sauce clings better to some. The student learned about Christopher Brown's work with the scanning laser microscope, and Christopher invited him to come to his lab. They found that spaghetti's functional performance is affected by the extruder die. Many manufacturers have switched to Teflon-coated dies to reduce dough friction and improve extruder throughput. But the spaghetti's surface, it turns out, becomes very smooth, and sauce won't cling to it as it would if a brass die is used. The gloss and color also are affected.
Surface texture has a tremendous impact on all types of foods. The roughness of a French fry influences the amount of oil that is absorbed during frying, for example. Oil uptake occurs at a micron level, and unless advanced microscopy is applied to analyze the surface texture, there is no way for a food engineer to know what the performance will be.
FE: So a Teflon-coated cutting surface could produce a healthier French fry that also is less expensive to fry because of reduced oil pickup.
Aguilera: That's right. Most oil inside the crust is sucked from the surface as the fries are removed from the fryer. Drainage would be facilitated and less oil would cling to cuts that are smoother in the micron range.
FE: Have you used a scanning laser microscope in your confectionery research?
Aguilera: Yes. After acquiring the images, we have applied fractal analysis to quantify the roughness of bloomed chocolate and other surfaces. Fractal analysis puts a number to complex geometries to develop a map of the exact kinetics at various temperatures and surface roughness. The geometric profile of a bloomed chocolate is not a straight line but a series of hills and valleys. I don't know if we'll ever develop a reduced calorie chocolate that tastes exactly like regular chocolate, but unless we know how the structure of chocolate is made and destroyed in the mouth, we're doomed to spend years on ingredient trial and error.
FE: What are some other instances where a better understanding of the microstructure of food impacts processing?
Aguilera: Artificial caviar is a concept that was patented many years ago but never successful commercially because of costs. But chefs are finding they can create artificial caviar by making a solution of a gelling polymer, adding flavor and colors and then using a syringe-type die to make droplets that form little balls of gel when they contact a calcium solution.
FE: Are you suggesting that the processing tools used today are inappropriate?
Aguilera: Nobody hammers a nail with a bulldozer, but often the dimensions of the key microstructural elements of a food are grossly out of proportion to the size of the device used to produce it. We make droplets in emulsions by dissipating a lot of energy in a 1 millimeter gap, but the structural element is only 10 microns, 100 times smaller. This will change. We are starting to use membranes with pores comparable to emulsion droplets, just as the cow does with milk.
FE: To what extent is the shift from a process engineering focus to product engineering occurring?
Aguilera: At the 2004 International Congress of Engineering and Food, thermal processing, mass transfer and other traditional approaches to food engineering were the focus of many of the papers, but the most popular topics were processing and characterization of structured polyphasic systems. These are complex, multicomponent mixtures with many phases, such as ice cream, which has fat, water, sugar, and at least three phases: solid ice, liquid sugar solution and gas air bubbles. Structured polyphasic systems weren't even being discussed at the first Congress in 1974.
Interfaces are occurring between scientific and culinary communities that never interacted before. Chefs are using centrifuges to create sophisticated new foods. Molecular biologists are creating tomatoes with stronger cell walls that can yield better paste. We're developing interfaces with the pharmaceutical industry for controlled release of flavors and nutritionals. By developing relationships with nutritionists, material scientists and others, we can design products to meet new consumer demands.
Looking for a reprint of this article?
From high-res PDFs to custom plaques, order your copy today!