Electric pulses keep beating
Commercial applications for pulsed electric fields are being approached a bit differently in the European food community than in the US.
Medical gallows humor is reflected in the adage, “The operation was a success, but the patient died.” The food equivalent for pulsed electric fields (PEF) is Genesis, a Portland, OR juice company that won FDA approval for PEF processing but became financially insolvent while waiting for it.
Rather than PEF’s death knell, Genesis was just a bump in the road to mainstream applications, champions of the technology insist. Commercial-scale development continues at Ohio State University, and Bedford, MA-based Diversified Technologies Inc. recently delivered a solid-state PEF generator to food scientists at Washington State University. Though gentle processing and preservation of food’s nutrients is PEF’s appeal, microbial inactivation is the primary focus of US research.
A different tack is being taken at DIL, the German Institute of Food Technologies, in Quakenbruck, Germany. For the last two years, Volker Heinz and Stefan Toepfl have built on work they did at the Berlin University of Technology. Instead of pasteurization or sterilization, they have focused on augmenting conventional processes, driving down energy demands, increasing yields and reducing the cost of their system. Off-the-shelf components and standardized parts are used whenever possible in engineering the solid-state el-Crack, a name borrowed from an ill-fated attempt to commercialize PEF in the 1980s.
Toepfl is DIL’s process development leader. He has authored several papers on the technology. Toepfl received his doctorate in food process engineering in 2006.
FE: When did you first become involved in PEF?
Toepfl: Seven years ago, when I was studying under Dr. Dietrich Knorr at the Berlin University of Technology. It was there that I met Volker Heinz, who has worked with PEF since 1992 and now is the head of DIL. The institute has a staff of 20 people dedicated to turning the potential in an idea into an industrial practice by uncovering the hidden costs and designing commercially affordable systems. In the last two years, we have been able to cut end-users’ investment costs in half. A 30 kV system now can be fabricated for about 125,000 euros, which doesn’t scare small and mid-sized companies as much.
FE: There are contradictions in the literature on PEF’s interaction with enzymes. Is the microbiology of PEF well understood?
Toepfl: It is well understood that various microbes display different resistance to electrical pulses. Yeast is more sensitive than smaller microorganisms, for example. What is less understood is the impact of pH and other factors on a wide variety of products. From our point of view, the mechanisms behind the reaction of enzymes to electrical pulses are unknown. Temperature gradation might have an impact, but some of the literature is contradictory. It’s very product specific. PEF isn’t always effective in enzymatic inactivation. On the other hand, this could be an advantage in some applications, such as foods containing eggs where you don’t want to impact the proteins.
FE: What does the el-Crack name mean?
Toepfl: It is short for electrical cracking of cells. The technology was developed in the 1960s and applied commercially, with ultrafiltration, in the 1980s by Krupp Maschinentechnik to extract oil in a fish slurry in Norway. Unfortunately, they didn’t have semiconductors and other technologies to keep the process stable. Nevertheless, PEF was shown to be effective, and in the early 1990s, researchers at Washington State, Ohio State and Berlin Universities renewed focus on the technology.
FE: The process chamber for Ohio State’s biggest unit is 1.2cm wide. How large is DIL’s chamber?
Toepfl: The largest currently is 6cm, and we could build one 10cm, no problem. If the goal was microbial inactivation, that would require a prohibitive amount of power, but we’re simply trying to destroy plant and animal cells to improve mass transfer. We can process up to 15 tons an hour of olives, meat and juices with particulate, and we’re building a prototype to treat pork haunches to improve drying or brining.
Cured hams hang for three months in a refrigerated room; by pretreating with PEF, the process can be speeded up by 25-30%, resulting in significant energy savings. Even a 10cm pipe isn’t big enough to treat a ham, so we’ve designed a conveying system with electrodes in rotating rows to pulse electrical waves. Currently it’s a batch system, but we’re focusing on developing a continuous process.
Just as destruction of the cell membrane helps accelerate water removal during curing, it accelerates water uptake during brining. Conventional processes rely on freezing and slow thawing, but that requires much more energy and time compared to PEF, which takes a few seconds.
FE: Ohio State’s system applies up to 40,000 volts per centimeter and 600 pulses per second. Is lower power a disadvantage in el-Crack?
Toepfl: Higher power aids in microbiological destruction, and their method of cycling energy input followed by intermediate cooling is good, but it’s not appropriate for all products. If you let the temperature of the product increase a bit and not cool it, synergies can occur. If a particular food can be treated at 40°C (104°F) without damaging it, why not? In the last year, we’ve identified how much temperature many products can withstand. The residence time is measured in seconds, and the thermal load is far below that of conventional processes.
FE: Is system maintenance an issue?
Toepfl: Five or six years ago, the electrodes were thought to be a problem, but that no longer is the case. Salt content and product pH affect the useful life; the more acidic, the more corrosion. But stainless steel construction has extended the life, and titanium could extend it further. In addition, the switch to bipolar pulses, where each positive pulse is reversed, has helped increase electrode life by a factor of 10. Electrodes last six months to a year or more, and replacement takes a few minutes.
FE: Your system makes use of a rectangular wave form. How does that impact performance?
Toepfl: Rectangular waves are less energy efficient than square waves, but the total energy inputted is a more significant factor. We focused on the cheapest way to generate power using standard components. The transformer takes 1-2 kW pulses and transforms them into high voltage in a separate cabinet that is protected with door locks and other safety defaults. The treatment chamber is fully encapsulated, and the five-electrode system is double grounded. You can touch it while it is operating without a problem. If you touched the cabinet when it is open, you would be killed, of course.
FE: What are some of the more promising applications for PEF?
Toepfl: We realized huge yield increases with apples in lab tests, though the increase was not that great in full-scale production. Carrot juice, on the other hand, is very promising. We were able to increase yield to 73% from 65% with conventional processes. Olive oil extraction also is a good application.
Experiments with sugar beets at Berlin Technical showed yield improvements, but it is best to focus on processes that have not been fully optimized yet and where there is a huge difference between theoretical yield and current commercial yield.
FE: What are the estimated treatment costs for various products?
Toepfl: For microbiological inactivation in a fully optimized unit, using heat in combination with pulse and recovering the heat in the chamber, we calculate the cost at one cent per liter. If PEF isn’t used in combination with heat, two to five cents per liter is realistic. For very heat-sensitive products that require active cooling, it rises to 10 cents a liter.
If the goal is aiding mass transfer of a juice product, one-tenth of a cent per liter is realistic. Plant cells are huge and easily broken, compared to animal cells, and cell size is a cost variable.

Stefan Toepfl, leader-process development, DIL (Deutsches Institut für Lebensmitteltechnik), the German Institute of Food Technologies, Quakenbruck, Germany
Rather than PEF’s death knell, Genesis was just a bump in the road to mainstream applications, champions of the technology insist. Commercial-scale development continues at Ohio State University, and Bedford, MA-based Diversified Technologies Inc. recently delivered a solid-state PEF generator to food scientists at Washington State University. Though gentle processing and preservation of food’s nutrients is PEF’s appeal, microbial inactivation is the primary focus of US research.
A different tack is being taken at DIL, the German Institute of Food Technologies, in Quakenbruck, Germany. For the last two years, Volker Heinz and Stefan Toepfl have built on work they did at the Berlin University of Technology. Instead of pasteurization or sterilization, they have focused on augmenting conventional processes, driving down energy demands, increasing yields and reducing the cost of their system. Off-the-shelf components and standardized parts are used whenever possible in engineering the solid-state el-Crack, a name borrowed from an ill-fated attempt to commercialize PEF in the 1980s.
Toepfl is DIL’s process development leader. He has authored several papers on the technology. Toepfl received his doctorate in food process engineering in 2006.
FE: When did you first become involved in PEF?
Toepfl: Seven years ago, when I was studying under Dr. Dietrich Knorr at the Berlin University of Technology. It was there that I met Volker Heinz, who has worked with PEF since 1992 and now is the head of DIL. The institute has a staff of 20 people dedicated to turning the potential in an idea into an industrial practice by uncovering the hidden costs and designing commercially affordable systems. In the last two years, we have been able to cut end-users’ investment costs in half. A 30 kV system now can be fabricated for about 125,000 euros, which doesn’t scare small and mid-sized companies as much.
FE: There are contradictions in the literature on PEF’s interaction with enzymes. Is the microbiology of PEF well understood?
Toepfl: It is well understood that various microbes display different resistance to electrical pulses. Yeast is more sensitive than smaller microorganisms, for example. What is less understood is the impact of pH and other factors on a wide variety of products. From our point of view, the mechanisms behind the reaction of enzymes to electrical pulses are unknown. Temperature gradation might have an impact, but some of the literature is contradictory. It’s very product specific. PEF isn’t always effective in enzymatic inactivation. On the other hand, this could be an advantage in some applications, such as foods containing eggs where you don’t want to impact the proteins.
FE: What does the el-Crack name mean?
Toepfl: It is short for electrical cracking of cells. The technology was developed in the 1960s and applied commercially, with ultrafiltration, in the 1980s by Krupp Maschinentechnik to extract oil in a fish slurry in Norway. Unfortunately, they didn’t have semiconductors and other technologies to keep the process stable. Nevertheless, PEF was shown to be effective, and in the early 1990s, researchers at Washington State, Ohio State and Berlin Universities renewed focus on the technology.
FE: The process chamber for Ohio State’s biggest unit is 1.2cm wide. How large is DIL’s chamber?
Toepfl: The largest currently is 6cm, and we could build one 10cm, no problem. If the goal was microbial inactivation, that would require a prohibitive amount of power, but we’re simply trying to destroy plant and animal cells to improve mass transfer. We can process up to 15 tons an hour of olives, meat and juices with particulate, and we’re building a prototype to treat pork haunches to improve drying or brining.
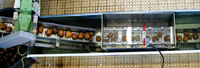
Just as destruction of the cell membrane helps accelerate water removal during curing, it accelerates water uptake during brining. Conventional processes rely on freezing and slow thawing, but that requires much more energy and time compared to PEF, which takes a few seconds.

Pulsed electric processing of solid foods is one of the areas being developed by German technicians. Pictured is a static unit for fruits and vegetables, while the diagram depicts a unit for continuous processing of up to 4,400 lbs. of meat an hour. Source: Deutsches Institut für Lebensmitteltechnik.
Toepfl: Higher power aids in microbiological destruction, and their method of cycling energy input followed by intermediate cooling is good, but it’s not appropriate for all products. If you let the temperature of the product increase a bit and not cool it, synergies can occur. If a particular food can be treated at 40°C (104°F) without damaging it, why not? In the last year, we’ve identified how much temperature many products can withstand. The residence time is measured in seconds, and the thermal load is far below that of conventional processes.
FE: Is system maintenance an issue?
Toepfl: Five or six years ago, the electrodes were thought to be a problem, but that no longer is the case. Salt content and product pH affect the useful life; the more acidic, the more corrosion. But stainless steel construction has extended the life, and titanium could extend it further. In addition, the switch to bipolar pulses, where each positive pulse is reversed, has helped increase electrode life by a factor of 10. Electrodes last six months to a year or more, and replacement takes a few minutes.
FE: Your system makes use of a rectangular wave form. How does that impact performance?
Toepfl: Rectangular waves are less energy efficient than square waves, but the total energy inputted is a more significant factor. We focused on the cheapest way to generate power using standard components. The transformer takes 1-2 kW pulses and transforms them into high voltage in a separate cabinet that is protected with door locks and other safety defaults. The treatment chamber is fully encapsulated, and the five-electrode system is double grounded. You can touch it while it is operating without a problem. If you touched the cabinet when it is open, you would be killed, of course.
FE: What are some of the more promising applications for PEF?
Toepfl: We realized huge yield increases with apples in lab tests, though the increase was not that great in full-scale production. Carrot juice, on the other hand, is very promising. We were able to increase yield to 73% from 65% with conventional processes. Olive oil extraction also is a good application.
Experiments with sugar beets at Berlin Technical showed yield improvements, but it is best to focus on processes that have not been fully optimized yet and where there is a huge difference between theoretical yield and current commercial yield.
FE: What are the estimated treatment costs for various products?
Toepfl: For microbiological inactivation in a fully optimized unit, using heat in combination with pulse and recovering the heat in the chamber, we calculate the cost at one cent per liter. If PEF isn’t used in combination with heat, two to five cents per liter is realistic. For very heat-sensitive products that require active cooling, it rises to 10 cents a liter.
If the goal is aiding mass transfer of a juice product, one-tenth of a cent per liter is realistic. Plant cells are huge and easily broken, compared to animal cells, and cell size is a cost variable.
Looking for a reprint of this article?
From high-res PDFs to custom plaques, order your copy today!